Nature Communications: motor adaptations & cerebellum
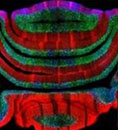
OUR MOTOR ADAPTATIONS ARE STORED IN THE CEREBELLUM
Adapting our movements following injury or intensive training is possible thanks to the processing and storage of a multitude of sensory, proprioceptive and motor information by the cerebellar cortex. By mapping the synaptic connections in the cerebellum of trained and injured mice, Dr Philippe Isope and his team (INCI, Strasbourg) in collaboration with Demian Battaglia’ team (LNCA, USIAS) have shown that the neural networks of the cerebellar cortex store sensorimotor adaptations specific to each individual. These results are published in the journal Nature Communications.
Graphical Abstract
Adapting our behaviour to our environment is essential for our survival. Adapting involves a large part of our brain. Indeed, the dynamics of neural networks in multiple brain areas, in particular the cerebellar cortex, process and combine data from the environment as well as proprioceptive information from our own body. These calculations are used to predict the outcome of our actions in order to anticipate or correct our movements and make them fast and efficient. To achieve this prediction, the researchers have hypothesized that the neural maps of the cerebellum store the coordinates of our muscles according to the motor context in which we are moving. The storage would be made possible by changes in the strength of the connections between cells in the cerebellar cortex, in particular the synapses between granule cells and Purkinje cells, which account for more than half of the synapses in the whole brain.
The scientists sought to test this hypothesis in mice in different locomotor contexts: when the animals are trained to gallop in a wheel, after altering their sciatic nerve, or during the development of walking in young animals. In these different contexts, they combined electrophysiological recordings with ex vivo photostimulation experiments and thus mapped the connections between granule cells and Purkinje cells in the region of the cerebellum specialized in gait adaptation. They describe for the first time that the spatial organization of synaptic connections is related to the activity of the animal and is specific to the locomotor context. The spatial organization of synaptic connections in the cerebellar cortex therefore encodes the locomotor context defining connectivity maps.
To be able to study the modifications of these maps in different motor contexts and to describe their spatial structure, which is neither completely disordered nor completely ordered, but complex, the researchers found the appropriate language for describing the complex 'shapes' of the maps: graph theory. This mathematical tool has already been successfully used to understand the complex network organization of very different systems such as ecosystems, the genome and the interactions between genes and proteins within a cell. In neuroscience, the language of networks has already been used to describe the 'connectome', or set of connections, between brain regions. Here, scientists identify the same level of complexity typical of these large-scale circuits within the connections formed by granular cells with individual Purkinje cells.
PI
Graph theory not only makes it possible to describe the overall structure of synaptic maps without anatomical assumptions, but also to extract complex cooperative relationships between the different sites of the maps. Thus, the connections between granular cells and a Purkinje cell form a patchwork of connected and silent areas, and the relationships between these areas form a specific structure of an individual's adaptation to the locomotor context. Surprisingly, the structural features of the synaptic maps, captured by their description derived from graph theory, allow for accurate prediction of how an individual behaves in a given locomotor context. The researchers show that synaptic connectivity maps encode features of movement in each specific context (e.g. limb movement during walking or running). Synaptic connectivity maps thus represent individual-specific engrams of adaptive behaviours established throughout life.
These results may allow the identification of functional markers of sensorimotor adaptation in the cerebellum and thus target more specific regions in brain stimulation protocols in cerebellum-dependent pathologies.